When everything in the universe changed

For millions of years after the Big Bang , after the universe's particle soup had cooled, the cosmos was a dark, dull place. There were no stars to make light. No swirling galaxies. No planets. And the entire universe was wrapped in neutral hydrogen gas .
Then, perhaps about 100 million years later, everything began to change. In the billions of years that followed, the universe transformed from a bland and unimpressive landscape into a rich and dynamic one. This profound change began when the first stars ignited. As they burned, generating heat and forging new matter, their intense light began to tear apart the hydrogen that pervaded the universe. Everywhere, electrons were stripped from these atoms, leaving most of the hydrogen—the most abundant element in the universe—in the ionized state where it remains today.
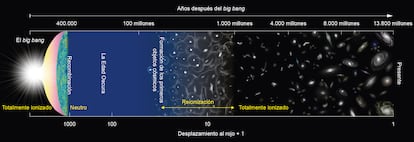
This crucial period, in which all that hydrogen transitioned from one form to another, is known as the epoch of reionization. It began with our cosmic dawn and gave way to the modern era with all its wonderful textures and features. It serves as a backdrop for when the universe grew.
"It's the last major change to occur in our universe," says theoretical astrophysicist Julián Muñoz of the University of Texas at Austin (USA). Everything changed in those billion years, and not much has changed in the billions of years since.
Although there are models that describe how this great transition could have occurred, major gaps remain. When did the first stars form, and when did the light escaping from their host galaxies trigger reionization? What types of galaxies were most responsible for this, and what role did black holes play? How did reionization occur over time and space? What clues can it provide to other cosmic mysteries, such as the nature of dark matter?
“We don’t understand how the universe came to be what it is today,” says Muñoz. Some answers are now within reach, thanks to new tools that allow scientists to peer into the depths of the universe’s first billion years. The James Webb Space Telescope (JWST), which launched in 2021, is observing galaxies that existed only hundreds of millions of years after the Big Bang and is already yielding surprises. At the same time, next-generation radio telescopes are focusing not on galaxies, but on the neutral hydrogen that once pervaded all space. That hydrogen provides clues about how the epoch of reionization and other features of the cosmos unfolded.
“The tools we can use now to study this era in cosmic history are unlike anything we've had before,” says astrophysicist Rob Simcoe of the Massachusetts Institute of Technology (MIT).
Light in abundanceOur current understanding of the development of the early universe goes something like this: after the Big Bang, 13.8 billion years ago, the cosmos expanded and the primordial soup of subatomic particles cooled. In the first second, protons and neutrons formed. In the first few minutes, they coalesced into atomic nuclei. About 380,000 years later, those nuclei began capturing electrons to form the first atoms. This milestone, in which the ionized soup turned into neutral atoms, is known as recombination (a misnomer, since nuclei and electrons had never combined before).
Until they were captured in atoms, free electrons scattered light like a dense fog in a car's headlights. But with electrons controlled, photons could be shot out into the cosmos. Today, those light particles reach us in the form of a faint glow known as the cosmic microwave background.
Then the universe entered what is known as the Dark Ages. With hydrogen and some helium in the cosmos, there was nothing that could produce light. However, bubbles of dark matter took to attracting surrounding gas, and some of it condensed enough to trigger nuclear fusion. A hundred million years or more after the Big Bang, the first stars ignited in our cosmic dawn. As these first stars burned, their ionizing ultraviolet light began to escape from their galaxies. This created bubbles of ionized hydrogen that grew until they coalesced, eventually filling the cosmos.
JWST is poised to answer many questions about the earliest galaxies and how their light drove the reionization process. For now, however, the telescope is yielding more questions than answers. In the early days, there were many more galaxies than scientists thought, and these galaxies produced far more light than was needed to reionize the universe.
The first images released by the telescope were packed with galaxies dating back less than 600 million years after the Big Bang. Then, in late 2022, came confirmation of the oldest galaxy to date: it existed just 350 million years after the Big Bang . That record was surpassed by astrophysicist Brant Robertson of the University of California, Santa Cruz, and his colleagues when they announced the existence of a galaxy dating back 290 million years to the Big Bang .
Many of these galaxies are brighter and more massive than expected: in 2023, six galaxies dating back 700 million years after the Big Bang made headlines for how mature they already appeared. Despite their early age, their stellar masses rival that of the current Milky Way, which has 60 billion solar masses of stars.
Standard theory can't explain so much star formation so early, which is why these galaxies were nicknamed the "universe-breakers." "It's absolutely crazy," says astrophysicist Erica Nelson of the University of Colorado at Boulder and co-author of the study. "It implies an early universe that is either more chaotic and burstier than we thought, or a universe in which things can evolve more rapidly."
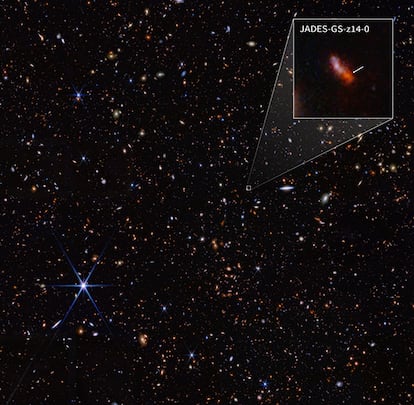
The discoveries could force a reexamination of galaxy evolution . And they raise big questions about reionization. Even the faintest primitive galaxies detected by JWST produce a large amount of reionizing light— four times more than expected , astrophysicist Hakim Atek of the Paris Institute of Astrophysics and his colleagues have discovered. Despite their low luminosity, there are enough of these galaxies to reionize the universe almost by themselves.
And JWST is also uncovering evidence that supermassive black holes formed much earlier in cosmic history than previously thought; the high-energy emissions they generate as they feed on surrounding matter would also have contributed to reionization.
With all that light, the universe should have reionized earlier than we know, Muñoz and colleagues suggest in a 2024 paper titled Reionization after JWST: A Photon Budget Crisis?
It's not really a crisis, says Muñoz. Existing research has established that reionization ended 1.1 billion years after the Big Bang. But the apparent overabundance of reionizing light is a clear sign that something is missing from our picture of the early universe. "We don't know all the pieces of the puzzle," he says.
Searching for clues in hydrogenOther efforts hope to track reionization using state-of-the-art radio telescopes to see how much neutral hydrogen existed over time in the early universe. Scientists have probed this hydrogen in other ways. The scattering of light from the cosmic microwave background, for example, offers clues about the total amount of reionization since that light was emitted, some 380,000 years after the Big Bang.
Quasars, the brilliant beacons of radiation produced by massive, feeding black holes, offer another clue. Neutral hydrogen absorbs specific wavelengths of light from quasars on their way to an observer, providing a signal of hydrogen's presence. But as we move toward earlier epochs, there are fewer quasars.
So scientists are now aiming to detect a radio signal originating from neutral hydrogen itself, before it ionized, stretching back to the dawn of space and even into the Dark Ages. This signal, known as the 21-centimeter line, has been detected since the 1950s and is widely used in astronomy, but it has not been definitively located since the early universe.
The radio signal arises due to a quantum transition in the electron of neutral hydrogen. The transition, which emits a small amount of electromagnetic radiation at a wavelength of 21 centimeters, does not occur often. But when neutral hydrogen is abundant, it can be detected.
And the signal can do more than just track the whereabouts of neutral hydrogen. It also serves as a kind of thermometer. Scientists can use it to better understand cosmic temperature, including clues about when energy is injected into the intergalactic medium in the form of light or heat.
These bursts of energy could come from the earliest stars and the black holes that feed them. Or the energy could indicate something more exotic: interactions between dark matter and itself, or unknown interactions between dark matter and more familiar matter. Such interactions, Muñoz notes, could heat or cool the intergalactic medium. The 21-cm line offers a way to probe the processes at play, including those stimulated by unexpected physics. “It can provide information that wouldn’t be obtained otherwise,” he says.
One telescope that's looking for this fingerprint is the Hydrogen Epoch of Reionization Array (HERA). While JWST is known for its complexity and cost, HERA is simpler. It's "made of PVC pipe, wire mesh, and telephone poles," explains astrophysicist Josh Dillon of the University of California, Berkeley.
HERA consists of 350 radio antennas spread across 5% of a square kilometer of South Africa's Northern Cape province. Although the telescope itself is low-tech, its observations require the most advanced signal processing and data analysis available. This is because the inherently weak signal must be detected amid the radio noise of our galaxy and others.
Dillon compares detecting the 21-centimeter signal to hearing the highs at a concert when the lows are 100,000 times louder. “That’s why it hasn’t been done yet,” he says.
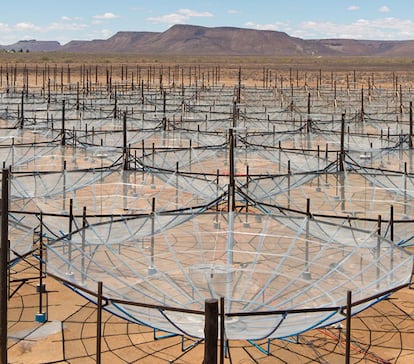
HERA seeks a statistical measure of the spatial fluctuations in the 21-centimeter signal. These fluctuations are due to variations in the distribution of neutral hydrogen in the cosmos and therefore provide an insight into how gas, stars, and galaxies are distributed. Other teams, however, aim to make a global measurement that captures an average signal across the entire cosmos. Since the techniques differ, one could help verify the other.
Dark matter has already been invoked to explain one purported detection . In 2018, researchers with the Experiment to Detect the Signature of the Epoch of Global Reionization, or EDGES, reported detecting the 21-centimeter-averaged signal corresponding to the moment when light from the first stars began interacting with the surrounding hydrogen.
The signal is stronger than expected, suggesting cooler hydrogen gas than anticipated, which has fueled widespread skepticism surrounding the claim . Some researchers have pointed to interactions between hydrogen gas and dark matter as a possible explanation, but such an explanation would require unexpected physics.
“There are a lot of fanciful theories,” says observational cosmologist Sarah Bosman of the University of Heidelberg in Germany. “They have to be fanciful,” she points out, because no ordinary physics would produce the power that EDGES saw.
Bosman admits to being one of the few people enthusiastic about the claim, which she says has motivated researchers to work on other experiments that could confirm or refute it. “It’s given this field a huge boost,” she says.
HERA and other telescopes are the precursors to the Square Kilometer Array, which will attempt to map the 21-centimeter signal across the entire sky. This array will connect radio antennas in South Africa and Australia to form the largest radio telescope ever built. Although still under construction, the telescope has connected two of its stations to collect its first data in 2024.
Better tools, deeper insightsNo one really knows what to expect from the 21-centimeter signal, Bosman notes. It might require only minor adjustments to the existing picture of cosmic evolution, or it might uncover new physics that completely rewrites our understanding. It's too early to tell.
But Dillon says the 21-centimeter line could one day offer “the largest data set possible.” The ultimate goal is to probe the time frame from about 100 million years after the Big Bang to a billion years later. That span represents less than 10% of the entire lifetime of the universe, but, due to the universe’s continued expansion, the time frame encompasses about half the volume of the visible universe.
Future instruments will help us peer into the past. There are several proposals for new radio telescopes in space and even on the Moon, where they would be free from terrestrial interference. The oldest 21-centimeter signal would reach us at wavelengths that are reflected by Earth's ionosphere, notes Anastasia Fialkov, a cosmologist and astrophysicist at the Institute of Astronomy in Cambridge, England. Space or lunar telescopes could avoid this problem.
Any 21-centimeter traces would be studied alongside JWST observations of the earliest galaxies, as well as observations from its successor, the Nancy Grace Roman Space Telescope, and future ground-based observatories such as the Extremely Large Telescope, a European initiative currently under construction in Chile.
Article translated by Debbie Ponchner .
This article originally appeared on Knowable en español , a nonprofit publication dedicated to making scientific knowledge accessible to everyone.
EL PAÍS